Synthetic biology: Bottom-up meets top-down
A step towards functionalizing artificial cells by finding a common language in engineering approaches
Scientists at the Max Planck Institute for Medical Research in Heidelberg, together with their collaborators at the ETH Zurich and at Heidelberg University, have taken a further step towards functionalizing artificial cell-sized compartments on the way to building a synthetic cell. They started from the changes in pH which resulted when a bacterial proton pump was illuminated, and used these to alter DNA origami structures and in turn to deform synthetic cells. Constructing complex sequences of transitions such as this may be important in creating synthetic cells with desired properties in the future. The results were published in Nature Communications on Friday, June 25th
Life as we know it arises from the complex interplay of its functional parts, leading to properties that distinguish living from non-living matter. Top-down and bottom-up synthetic biology have developed methods for building and manipulating living systems. Both fields face challenges but both also present opportunities. For example, top-down synthetic biology has equipped existing cells with new functionalities, while the bottom-up approach starts with cellular parts to study their function in isolation. “Combining the possibilities of both approaches may be a key to engineering and programming living matter. We just need to find a common language,” says Kevin Jahnke, first author and PhD student in the Biophysical Engineering of Life Research Group at the MPI for Medical Research.
A recent publication describes the use of proton gradients to find a common language and construct a complex reaction pathway in a synthetic cell. The authors engineered light-harvesting bacteria (E. coli) to express the light-driven proton pump Xenorhodopsin, which increases the pH of the extracellular space when illuminated. The bacteria were then encapsulated in droplets, as a first step towards establishing light-triggered pH changes in a compartment. A DNA strand sequence that forms a triplex at low pH but is open at high pH was then synthesized. When the strand was tagged with cholesterol, the transition resulted also in attachment to the periphery of the compartment, as could be directly observed on illumination.
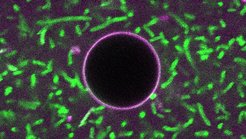
To further amplify this light-induced change in order to produce a visible change in morphology, giant unilamellar vesicles (GUVs) were used instead of droplets, and a ‘DNA origami’ (a larger organelle-like structure with programmable form, built using DNA technology) was added to the DNA strand. The overall system, comprising bacteria, GUVs, switchable DNA strands and DNA origami, now indeed showed changes of the GUVs from their spherical form in response to illumination. Due to the change in pH after activating the proton pumps with light, DNA origami attaches to the membrane of the GUV, where it polymerizes to deform the synthetic cell. The bacteria can thus communicate with the synthetic cells to adapt their shape.
This success strikingly illustrates the possibilities of using sequential structural transitions and complex reaction pathways to modify the behaviour of synthetic cells.
How does Kerstin Göpfrich, group leader at the MPIMR, view future research of her group? “Ultimately, we hope that constructing complex reaction pathways like these and integrating more functional modules may lead to synthetic cells with emergent properties. One day, a synthetic cell may be more than the sum of its parts.”