Introduction to Muscle Contraction, Part 2
Movement of the lever arm
Studies of the cross-bridge movement were undertaken by time resolved studies of contracting frog muscle using low angle x-ray fibe diffraction (Huxley et al. 1981; Irving et al. 1992). While these results are fully consistent with the swinging cross-bridge theory, the complexity of the system and low resolution of the method precludes an unambiguous interpretation. Therefore a simpler model system has been studied - decorated actin - an actin filament with a myosin cross-bridge bound to each actin. While the structure of decorated actin in the rigor state (no bound nucleotide) has been extensively studied (Moore et al. 1970) (Milligan and Flicker 1987) corresponding studies in the presence of ATP are difficult since the binding of ATP leads to rapid dissociation of the cross-bridges from actin.
Time-resolved electron micrograph studies in fact show no bulk change of the cross bridge orientation on binding ATP before dissociation takes place(Pollard et al. 1993) whereby a reorientation of the lever arm would not have been detected at the resolution attainable. High resolution electron micrographs of actin decorated with smooth muscle myosin, however, show a 30-35° rotation of the lever arm on binding ADP (Jontes et al. 1995; Whittaker et al. 1995). Although the main movement of the lever arm would be expected to be associated with phosphate release since this is a step associated with a large change in free energy, some fraction of the movement could arise from ADP binding and release. Moreover, this movement should be recoverable on adding ADP to actomyosin, which indeed it is. Although the effect has only been found in smooth muscle myosins it is generally important in providing the first direct demonstration of a nucleotide-induced lever-arm swing. More recent work using a spin label attached to a light chain also supports this result (Gollub et al. 1996). Moreover, x-ray diffraction studies of muscle fibres loaded with exogenous smooth muscle cross-bridges show the predicted changes in the fibre diffraction pattern resulting from the lever arm swing on binding ADP (Poole et al. 1997).
Purified myosin cross bridges (S1) can be attached to a substrate and used to transport actin filaments in vitro in the presence of ATP. A study by Spudich et al (Uyeda et al. 1996) shows that the speed of actin transport in motility assays is proportional to the length of the lever arm. Moreover, the fulcrum appears to lie near the broken helix (gg690-710) which contains the especially reactive thiols (SH1, SH2) of myosin. A similar result has been obtained by Manstein and coworkers using a synthetic lever arm made from repeating α-actinin repeats in place of the light chain binding region (Anson et al. 1996).Mutagenesis studies also indicate the importance of the SH1-SH2 region in controlling movement of the lever arm (Patterson and Spudich 1996). A ggG699A mutation, between the SH1 and SH2 groups, slows myosin transport of actin 100-fold (Kinose et al. 1996).
Specific fluorescent markers attached to the regulatory light chain show a small angular movement on contraction (Allen et al. 1995; Irving et al. 1995), whereas the lever arm hypothesis expects about 50° rotation. However, if only a fraction (ca. 10-20%) of the cross bridges in active muscle take part in contraction at any one time, the magnitude of this apparent rotation can be proportionally scaled up towards the anticipated value.
The Myosin Cross Bridge has two Confirmations
According to the Lymn-Taylor scheme, the myosin cross bridge would be expected to have two discernible conformations: (1) when it first attaches to actin with the products of hydrolysis still bound with the lever at the beginning of the working stroke; and (2) at the end of the working stroke when the phosphate and ADP are released. This sequence is often referred to as the power stroke. The end state is referred to as rigor, since it is the state muscle enters on ATP depletion. It is also called strong because it binds to actin quite tightly. The initial state is called the weak binding state because of its low affinity for actin (see Geeves and Conibear, 1995). We might anticipate that these two states of the myosin cross bridge might exist independently from actin and indeed protein crystallography shows this to be the case.
The chicken S1 structure was solved without bound nucleotide. Furthermore, the chicken S1 crystal structure fits excellently into the electron micrograph reconstructions of the strong actin-myosin nucleotide-free interaction (decorated actin). Therefore the crystal structure of chicken S1 would appear to represent the end of the power stroke or rigor state.
In addition, Rayment et al have studied a crystalline fragment of the dictyostelium myosin II cross-bridge which has been truncated after residue 761 (equivalent to gg781). The truncation eliminates the lever arm and the associated light chains (which aids crystallization). However, the converter domain is still present. The crystal structures of the 761 construct have been determined with a number of ATP analogs, particularly ADP.BeFx (Fisher et al. 1995) and ADP.vanadate (Smith and Rayment 1996). ADP.vanadate complexes are used as analogs of the transition state or possibly of the ADP.π state.
While the ADP.BeFx state looks similar to rigor, the ADP.vanadate structure shows, compared to the chicken rigor structure dramatic changes in shape of the S1 structure, There is a closing of the 50K upper/lower domain cleft, particularly around the γ-phosphate binding pocket, and large movements in the C-terminal region. The 50K upper/lower domains rotate a few degrees w.r.t. each other around the helix gg648-666 in a way which closes the nucleotide binding pocket (Fig 5) - a movement of some 5Å. At the same time the outer end of the long helix (the so called switch 2 helix, residues gg475-507) bends out 24° at residue V497. This is coupled to a rotation of the converter domain (gg711-781) by 70°. The fulcrum is provided by the mutual rotation of the distal part of the SH1-SH2 helix around the distal part of the switch 2 helix.

© Kenneth C. Holmes
Figs 6 and 7: A model of this new state is shown in Fig. 6. The coordinates of the missing lever arm have been generated from the chicken coordinates by superimposing the converter domains. For comparison Fig. 7) we have generated the corresponding diagram from the coordinates with ADP.BeFx bound in the active site(Fisher et al. 1995) which, for reasons stated below, we take to be the ADP state. On comparing Figs. 6 and 7 one sees that the end of the lever arm has moved through 12nm along the actin helix axis, which is greater than most estimates of the size of the power stroke. Therefore, it would appear that the ADP.vanadate state is indeed a model of the anticipated "beginning of power stroke" state. The mechanism for coupling the movement of the lever arm with the status of the nucleotide binding pocket revealed by this structure suggests that the two events are tightly coupled: pocket closed, lever up (beginning); , pocket open, lever down (end).
© Kenneth C. Holmes
A model of this new state is shown in Fig. 6. The coordinates of the missing lever arm have been generated from the chicken coordinates by superimposing the converter domains (for comparison Fig. 7), we have generated the corresponding diagram from the coordinates with ADP.BeFx bound in the active site (Fisher et al. 1995) which, for reasons stated below, we take to be the ADP state. On comparing Figs. 6 and 7 one sees that the end of the lever arm has moved through 12nm along the actin helix axis, which is greater than most estimates of the size of the power stroke. Therefore, it would appear that the ADP.vanadate state is indeed a model of the anticipated beginning of power stroke state.
The mechanism for coupling the movement of the lever arm with the status of the nucleotide binding pocket revealed by this structure suggests that the two events are tightly coupled: pocket closed, lever up (beginning) - pocket open, lever down (end).
This state could be influenced by the missing light chains
Since the experiments on dictyostelium myosin have used a truncated form, Smith and Rayment have urged caution in interpreting the results incase they should be caused in some way by the absense of the light chains or effects of crystal packing. In addition, the converter domain is partly disordered. Infact, the model building shows no conflict between the essential light chain and the N-terminal part of the myosin heavy chain. Moreover, a sequence comparison between 80 different myosin sequences (Cope et al, 1996) shows that there is a core myosin sequence which terminates exactly at the point where Smith and Rayments truncation occured. Beyond this point myosin sequences vary according to the number and type of light chains bound. Therefore, it would appear that there is a good chance that the results with bound ADP vanadate are to be taken at their face value. Moreover, an independent determination of the ADP.BeFxstructure (see below) shows very similar effects to those found with ADP.vanadate although the crystal packing is quite different.
For ATP-hydrolysis the 50k upper/lower domain cleft should close
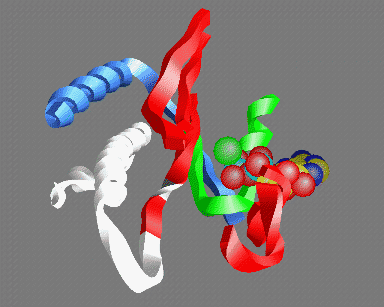
© Kenneth C. Holmes
Fig 5: A view of the ATP binding site looking out from the actin helix. Shown are: the P-loop (green); an MgATP molecule with the base at the back and the three phosphate groups in the front (carbon yellow, nitrogen blue, phosphate light-blue, oxygen red, magnesium green); parts of the 50K upper domain (red) including the so called "switch-1" region (right); the switch-2 region in the open "ATP" (white) and closed "ADP" (gray) conformations - the conserved glycine (gg466) is shown in gray or white- note that this residue moves about 5Å between the two conformations; the helix (gg648-666) (which acts as fulcrum for the relative rotation of the 50K upper and lower domains (blue)
© Kenneth C. Holmes
Smith and Rayment (Smith and Rayment 1996) point out the similarity of the active site of myosin in the closed form with ras p21 and other G-proteins and that the enzymes probably share a common mechanism for hydrolysis (Schweins et al. 1995). The differences between the open and closed forms of the myosin cross-bridge in the neighborhood of the active site reside almost entirely in the conformation of the linker region (gg465-470) which joins the 50K upper and lower domains. Smith and Rayment point out that this region is structurally equivalent to the so-called switch 2 region in ras p21 with which it also has a very strong sequence homology.
The mutual rotation and closing of the 50k upper/lower domains cleft causes this region to move by about 5Å. In the chicken crystal structure (open), which has no bound nucleotide and should therefore be in the rigor conformation, the switch 2 region is pulled away from the nucleotide binding pocket. A similar movement of the switch-2 region depending on whether di- or tri-nucleotide is bound is also found in the G-proteins. Only in the closed form (ADP.vanadate) can the hydrogen bond between the carbonyl of ggG466 and the γ-phosphate (Fig 5), which is an invariant characteristic of the G-protein active sites, be formed. Because of the importance of ggG466 (and other residues) for hydrolysis it is difficult to see how hydrolysis can proceed in the open (rigor) form which would therefore appear not to be an MgATPase: the closing would appear to be essential for hydrolysis.
ADP.BeFx can produce both open and closed states
ADP.BeFx is thought to be an analogue for ATP. Fisher et al (Fisher et al. 1995) solved the structure of dictyostelium S1 truncated at 761 with ADP.BeFx bound in the active site and found it to be remarkably similar to chicken S1 without nucleotide. This result appears to show that the structure of the ATP state is open, which is puzzling since it would not be able to hydrolyze the ATP. Moreover, the attitude of the converter domain (and hence the neck) is close to rigor which is also unexpected for the ATP state (c.f. Figs. 6 and 4). More recently Schlichting et al (Schlichting et al. 1997) have solved the structure of an ADP.BeFx complex of truncated S1 and find it to be essentially identical to the ADP.vanadate complex.
The active site is closed and the converter domain is in the rotated configuration. The construct used in this case was truncated at position 754 and is therefore 7 residues shorter than that used by Fisher et al. This results in a tighter binding of ADP (Kurzawa et al. 1997). Apparently, on account of this difference, in the shorter construct the binding energy of ADP.BeFx is adequate to tip the scales for the closed structure, whereas in the longer construct it was not. Therefore one can picture the transition between the two forms of myosin as being sensitively poised (but well determined - intermediate states have yet to show up): the structure solved by Fisher et al apparently corresponds to the ADP-bound state whereas the structure solved by Schlichting et al corresponds to the ATP-bound state. Comparing the Fisher et al ADP.BeFx state (Fig 6) with chicken rigor (Fig 4) there is in fact a 10° movement of the lever arm. This may reflect small changes in the angle of the lever arm induced by the binding of ADP but it could also reflect species differences.
Phosphate release
Actin binds to the open form of the 50k upper / lower cleft and thereby facilitates phosphate release.
The closed structure found with the ADP.vanadate generates a tight hydrogen bonding pattern for the γ-phosphate which probably explains the high phosphate affinity. This interaction in turn is important for stabilizing the closed form. Opening the cleft destroys the γ-phosphate binding pocket. Energy-filter cryo electron microscopy of decorated actin (Schröder et al. 1997) shows that the cleft may be open in the actin-myosin complex. Therefore it seems very likely that actin binding opens the cleft rather than closes the cleft as was suggested earlier (Rayment et al. 1993). Opening the cleft destroys the phosphate binding site and facilitates γ-phosphate release by a different route to that by which it entered (a "back door enzyme" - Yount et al. 1995).
Although kinetic studies provide evidence that the actin myosin binding in the presence of nucleotide is a multi-step process, there is no structural data on an initial weak binding of the closed form to actin. A consistent scheme may be developed by postulating that there is an additional transitory state, a bent closed form. We suppose that actin binds myosin with one main set of contacts at approximately constant geometry, namely as is seen in the rigor actin-myosin complex (i.e. the open form of myosin). The 50K lower domain probably forms the invariant contacts to actin: the switch from weak to strong binding probably involves the recruitment of loops (the 50K- 20K loop and the 404 loop) from the 50K upper domain to form the strong binding state. When confronted with myosin in the closed form actin probably binds the 50K lower domain first, which binds actin weakly. The subsequent binding of the loops produces an open form which releases the γ-phosphate and binds actin strongly.
Summary
Crystallographic studies show two distinct structural states for myosin S1: the open or end conformation which is characterized by the absence of nucleotide (rigor); and the closed or beginning state, which is favored by binding ATP or the products complex (ADP.Π). Myosin transports actin by switching between these two states. Open and closed refer to the status of the ATP binding site which extends from the 50K upper domain across to the 50K lower domain. This in turn is coupled to the rotation of a C-terminal lever arm. In the closed form the lever arm is at the beginning of the power stroke whereas in the open form it is at the end of the power stoke. The preference for open or closed is also controlled by binding to actin. We hypothesize that the closed state binds only weakly to actin. On this basis we can correlate the structural states with the Lymn-Taylor cycle.
Starting from an actin-myosin complex at the end of the power stroke, the binding of ATP brings about rapid closure of the cleft and concomitant release from actin. The closed state hydrolyses ATP to ADP.? without attaching to actin. Thereafter, the rebinding of myosin in the closed or beginning conformation of the products complex to actin opens the cleft to facilitate release of the ?-phosphate. Release of phosphate induces an isomerisation to the open end conformation since it is the presence of the ?-phosphate which stabilizes the closed form. The isomerisation results in large changes of angle of the lever arm (at the distal part of the myosin head). Since the S1 is strongly attached to actin at this stage this results in a 12nm transport of actin past myosin.